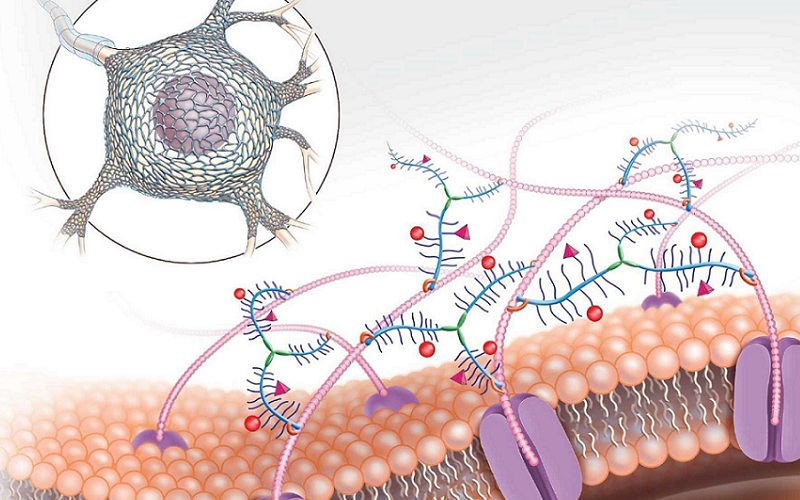
The human brain, a marvel of nature, is a vast universe of neurons, synapses, and intricate pathways. Each component, no matter how tiny, plays a crucial role in how we think, feel, remember, and interact with our surroundings. While many aspects of this complex organ have been studied and understood, there remain hidden gems of knowledge that continue to astonish researchers and enthusiasts alike. One such fascinating component is the “Perineuronal Net” or PNN. These microscopic structures, often overlooked, serve as both a guardian shield for our neurons and a regulator for our memories.
Contents
What are Perineuronal Nets (PNNs)?
The intricate landscape of our brain, replete with countless neurons and their associated structures, is a testament to nature’s complexity. Among these, the Perineuronal Nets (PNNs) might not be the most famous, but they are undoubtedly significant.
Definition and Basic Structure of PNNs
At their core, PNNs are specialized extracellular matrix structures that envelop certain neurons. Imagine a delicate meshwork, almost like a protective cocoon, wrapped around the neuron’s body and its dendrites (but not typically around the axon). This “cocoon” consists primarily of molecules known as chondroitin sulfate proteoglycans, along with other proteins and sugars. It’s this unique composition that gives PNNs their distinctive properties and functionalities.
Distribution in the Brain: Where Can PNNs Be Found?
PNNs aren’t randomly scattered throughout the brain; instead, their distribution is selective. They predominantly surround a particular type of neuron called the “fast-spiking parvalbumin-expressing interneurons.” These neurons are critical for processing information and play a pivotal role in controlling the activity and synchronization of neural circuits.
Interestingly, while PNNs are most commonly associated with the cerebral cortex (the brain’s outer layer responsible for many higher-order functions), they can also be found in various other regions, such as the amygdala (involved in emotion processing) and the thalamus (a relay station for sensory and motor signals). This selective distribution underscores their importance in modulating specific neural processes and pathways.
In essence, PNNs act as both protective barriers and functional modulators for some of the brain’s most crucial neurons, reinforcing the significance of understanding their role in the vast neural network.
Historical Context: Discovery of PNNs
The journey of scientific discovery is a winding path, marked with moments of serendipity, insight, and persistence. PNNs, despite their significance in modern neuroscience, weren’t always at the forefront of brain research.
Early Observations and Studies of PNNs
The story of PNNs dates back to the late 19th century. Using the primitive staining techniques available at the time, early neuroanatomists began noticing unusual patterns around certain neurons. These patterns, although faint and often inconsistent with the rudimentary dyes, hinted at the existence of a previously uncharted structure. However, due to the limitations in microscopy and biochemical techniques of that era, these observations remained largely anecdotal, and a definitive understanding eluded researchers [1].
Evolution in the Understanding of PNNs’ Role
The mid-20th century brought with it a revolution in both technology and molecular biology. Advanced staining techniques, electron microscopy, and the rise of molecular biology unveiled the elusive PNNs in clearer detail. Researchers could now discern their distinct mesh-like structure and began to hypothesize their potential protective role.
By the late 20th century and early 21st century, the field witnessed a surge in interest surrounding PNNs. Groundbreaking studies began to link these structures to various neural functions, especially in the context of plasticity and memory formation. Moreover, with the advent of sophisticated tools like proteomic analysis, scientists started to decode the intricate molecular composition of PNNs, offering insights into their multifaceted roles.
It’s a testament to the ever-evolving nature of science that what began as mere faint outlines in the late 1800s, transformed into one of the most intriguing focal points of contemporary neuroscience. PNNs, once the unsung heroes of the neural landscape, now stand as critical players in our understanding of brain function and health.
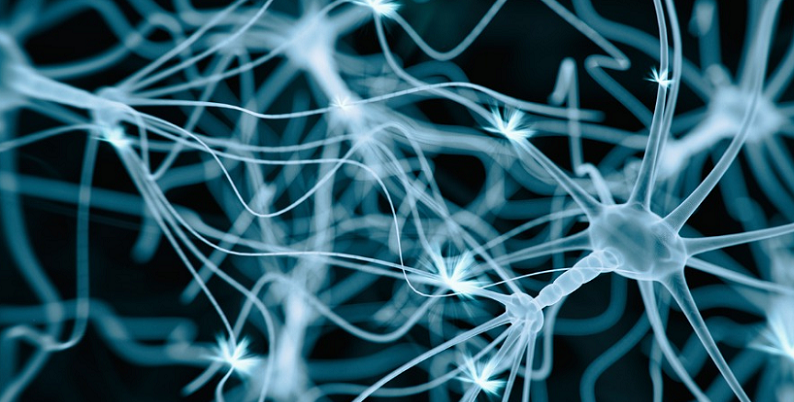
Role of PNNs in Protecting Neurons
The brain, an organ of profound complexity, is constantly at risk from both external and internal threats. Just as our skin acts as a barrier to protect our inner organs, neurons too need a safeguarding mechanism, particularly given their irreplaceable nature. This is where the perineuronal nets (PNNs) come into play. Their significance extends beyond being mere anatomical structures; they serve as the first line of defense, ensuring the health and stability of neurons. But how exactly do they achieve this protective role?
Physical Protection: The Meshwork
The name ‘net’ in PNNs isn’t just metaphorical; these structures literally form a meshwork around neurons. But why is this meshwork crucial?
Composition of PNNs: Chondroitin Sulfate Proteoglycans
PNNs are primarily composed of chondroitin sulfate proteoglycans, which are large molecules consisting of a protein core with attached sugar chains. This unique combination makes PNNs both flexible and sturdy. Much like a safety net under a trapeze artist, this meshwork can absorb external shocks or pressures, ensuring the neuron remains undamaged [2].
How This Structure Provides Protection
The dense nature of PNNs acts as a formidable barrier against potential threats. For instance, harmful molecules that could damage the neuron find it challenging to penetrate this mesh. Moreover, the PNNs’ structure aids in maintaining the neuron’s shape, preventing any undue stretching or compression, which could impede its functionality.
Protection from Neurochemical Changes
Beyond the physical shield they provide, PNNs also play an active role in maintaining the delicate neurochemical balance essential for a neuron’s health.
Role in Shielding from Oxidative Stress
Oxidative stress, a result of an imbalance between free radicals and antioxidants in the body, can be particularly harmful to neurons. PNNs have been shown to shield neurons from this stress, possibly by trapping antioxidants or by acting as a barrier against free radicals [3].
Buffering Excess Neurotransmitters
Neurotransmitters are the chemical messengers of the brain, and their balance is vital for proper neural function. PNNs can help regulate neurotransmitter levels in the synaptic cleft (the gap between two communicating neurons). By binding to excess neurotransmitters, PNNs ensure they don’t overstimulate the neuron, thereby preventing potential damage.
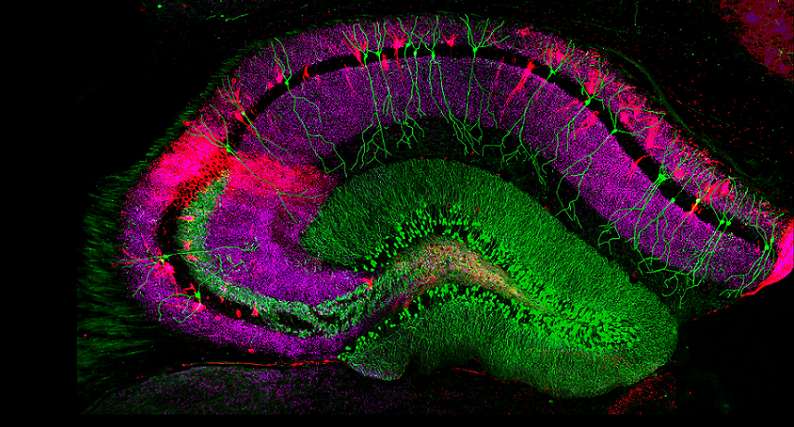
PNNs and Memory Regulation
While the protective role of PNNs is undeniably crucial, their involvement doesn’t end there. The brain is not just a static organ but a dynamic entity, continuously learning, adapting, and evolving. At the heart of this dynamism lies memory – our ability to store, retrieve, and modify information. PNNs, in their enigmatic ways, influence this very process.
Influence on Synaptic Plasticity
Synaptic plasticity is the brain’s ability to strengthen or weaken synapses (connections between neurons) based on experience. It’s the foundation of learning and memory. But how do PNNs play into this?
Critical Periods in Neural Development
Early in life, our brain undergoes ‘critical periods’ – specific windows of time when it’s highly receptive to external stimuli and can modify its structure and function accordingly. PNNs have been linked to the closure of these periods. As PNNs mature and solidify around neurons, they restrict the extent of synaptic modification, ensuring stability after learning has occurred [4].
Role in Solidifying Synaptic Connections
Beyond critical periods, PNNs play a continuing role in determining synaptic strength. By influencing the milieu around synapses, they can promote the retention of specific synaptic connections, effectively making certain memories more robust and long-lasting.
Impact on Long-Term Potentiation (LTP)
One of the most studied mechanisms for memory formation is Long-Term Potentiation (LTP) – a long-lasting enhancement in signal transmission between two neurons after repeated stimulation.
LTP: The Molecular Mechanism for Memory
At its core, LTP represents the cellular basis for learning. When two neurons are activated synchronously, the connection between them strengthens, making future activations more likely. This enhanced connection effectively stores a memory.
How PNNs Facilitate or Inhibit LTP
PNNs have a dual role in LTP. On one hand, by stabilizing synaptic connections, they can ensure that the strengthened synapses (post-LTP) remain robust. Conversely, their presence can also limit the extent to which LTP can occur by restricting synaptic modifications. This delicate balance ensures both memory stability and flexibility [5].
Possible Links to Memory Disorders
The intricate dance between PNNs and memory has also drawn attention in the context of memory disorders.
Degradation of PNNs in Age-Related Diseases
Studies have shown that in neurodegenerative diseases like Alzheimer’s, the integrity of PNNs is compromised. This degradation might contribute to the memory deficits characteristic of such conditions.
Potential Therapeutic Interventions Targeting PNNs
Given their pivotal role in memory, PNNs are being explored as potential therapeutic targets. By modulating PNNs, researchers hope to either enhance memory formation or combat memory degradation in various disorders.
References
[1] The roles of perineuronal nets and the perinodal extracellular matrix in neuronal function
[2] Casting a Wide Net: Role of Perineuronal Nets in Neural Plasticity
[3] Perineuronal Nets: Plasticity, Protection, and Therapeutic Potential
[4] Perineuronal nets protect long-term memory
[5] Is loss of perineuronal nets a critical pathological event in Alzheimer’s disease?




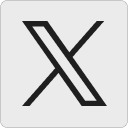




