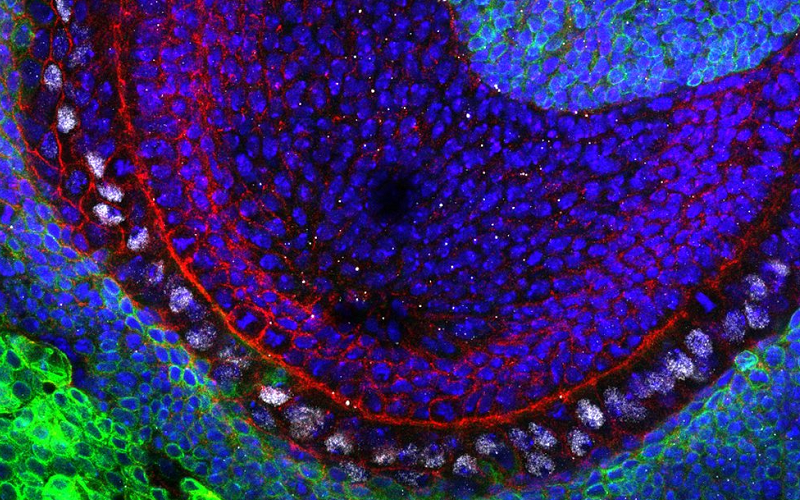
Neural stem cells and epigenetics represent a complex and emerging field of study that holds significant promise for our understanding of the brain, potential treatments for neurological disorders, and the broader field of regenerative medicine.
Neural stem cells, the self-renewing, multipotent cells that generate the main cell types of the nervous system, are key players in brain development and maintenance. Epigenetics, the study of changes in gene expression that do not involve alterations to the underlying DNA sequence, adds another layer of complexity to the biological functioning of these cells. How these two fields intersect, and the epigenetic tools and therapies being developed as a result, are reshaping our understanding of the brain’s inner workings.
Contents
Introduction to Neural Stem Cells and Epigenetics
The intricate world of neuroscience has been a subject of fascination and intense study over the years. One of the most intriguing aspects of this field is the role played by neural stem cells, a type of cell that is critical for the functioning and regeneration of our nervous system. Equally fascinating is the realm of epigenetics, which explores how gene expression changes without altering the DNA sequence. Together, these two areas of study offer a glimpse into the complex and awe-inspiring machinery that governs our bodies and minds.
Brief Overview of Neural Stem Cells
Neural stem cells are special types of cells found in our nervous system. These cells possess a unique characteristic that sets them apart from others — they are self-renewing and multipotent. This means that they have the ability to produce more of their own kind, as well as differentiate into various types of neural cells, such as neurons, astrocytes, and oligodendrocytes. The existence of neural stem cells was confirmed in the late 20th century, revolutionizing our understanding of brain development, maintenance, and its potential for regeneration.
Introduction to Epigenetics
While genetics provides the blueprint for the development and functioning of an organism, it doesn’t explain everything. Enter epigenetics, a field of study that examines changes in organisms caused by modification of gene expression, rather than alteration of the genetic code itself. Epigenetic changes can be caused by various factors such as age, environment, lifestyle, and disease state. These changes are heritable and have the potential to greatly influence an individual’s health and development.
The Connection Between Neural Stem Cells and Epigenetics
Epigenetics plays a pivotal role in controlling the fate of neural stem cells. Various epigenetic mechanisms, including DNA methylation and histone modification, can regulate gene expression in these cells, influencing their ability to renew themselves or differentiate into specific neural cell types[1].
This delicate balance of self-renewal and differentiation is what maintains the brain’s homeostasis and its ability to adapt and repair. Unraveling this complex interplay of neural stem cells and epigenetics is vital for understanding brain development and function, and has the potential to open new avenues for treating a variety of neurological disorders.
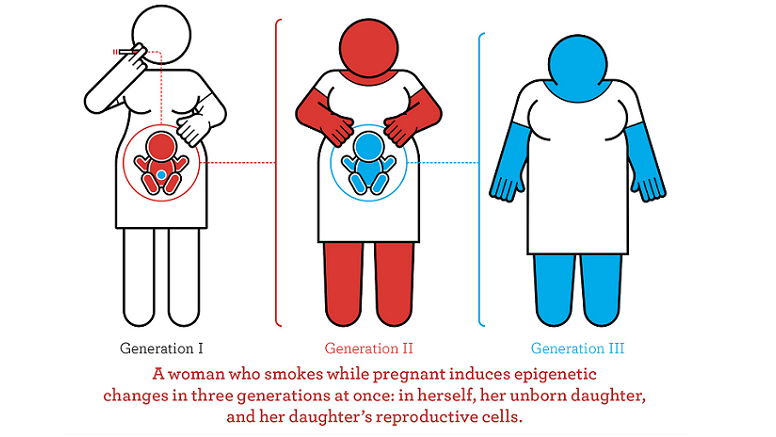
Understanding Neural Stem Cells
To truly appreciate the significance of the intersection between epigenetics and neural stem cells, it’s crucial to first delve deeper into the nature, function, and importance of these unique cells. They are, in many ways, the foundation upon which our understanding of the nervous system is built.
Defining Neural Stem Cells: An Overview
Neural stem cells are a unique kind of cell found within the nervous system. Unlike most cells in the body, they have two distinct abilities. First, they can self-replicate, meaning they can divide and create more neural stem cells. Second, they are multipotent, meaning they can differentiate, or turn into, several different types of cells found within the nervous system. This includes neurons, which transmit information throughout the body, and glial cells, like astrocytes and oligodendrocytes, which support and protect neurons.
Function and Importance of Neural Stem Cells
The dual capabilities of self-renewal and differentiation make neural stem cells critical for the development, maintenance, and repair of the nervous system. During early development, they generate the diverse range of cells that make up the brain and spinal cord.
In certain regions of the adult brain, such as the hippocampus and olfactory bulb, neural stem cells continue to produce new neurons in a process called neurogenesis, contributing to learning, memory, and mood regulation. Furthermore, their potential for regeneration also opens up the possibility for repairing the nervous system after injury or during degenerative diseases.
Neural Stem Cells and the Human Brain: An Integral Relationship
The relationship between neural stem cells and the human brain is indeed intricate. It’s not just about the cells themselves, but how they interact with each other, their environment, and, most interestingly, how they react to various genetic and epigenetic factors[2].
As mentioned earlier, in certain regions of the adult brain, these stem cells continue to divide and differentiate, contributing to the brain’s plasticity — the ability to adapt and reorganize itself. This process is influenced by a multitude of factors, including neural activity, aging, stress, and disease.
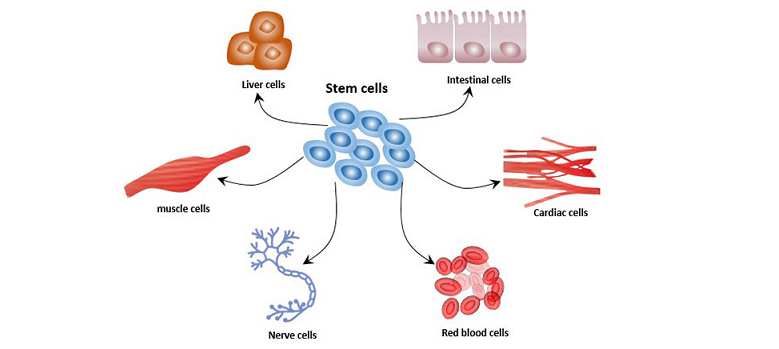
The Power of Epigenetics
Having explored the world of neural stem cells, we now turn our attention to the equally intriguing and complex field of epigenetics. Epigenetics brings an added layer of control and refinement to the workings of our genes and, by extension, the functionalities of our cells. Its influence extends to every facet of our biology, from development to aging, and has immense implications in health and disease.
What is Epigenetics: Simplifying the Complex Concept
Epigenetics, derived from the Greek word ‘epi’ meaning ‘on top of’, refers to changes in gene expression that occur without any alterations in the underlying DNA sequence. Essentially, while our DNA provides the basic instructions for life, epigenetic modifications decide which genes are turned ‘on’ or ‘off’ at any given time[3].
It’s akin to having sheet music for an entire orchestra — while the notes for every instrument are all there, the conductor, or the ‘epigenetic factors’, decide which instruments play, when they play, and how loudly or softly they play. These subtle adjustments create the harmonious symphony of life as we know it.
The Role of Epigenetic Changes in Gene Expression
Epigenetic changes can have significant effects on gene expression. They do this primarily through two mechanisms: DNA methylation and histone modification. DNA methylation typically acts to repress gene expression. When a molecule called a methyl group is added to the DNA strand, it can inhibit the machinery that reads the DNA, effectively turning off the gene.
Conversely, histone modifications can either enhance or repress gene expression. Histones are proteins around which DNA is wrapped, and changes to these proteins can affect how tightly or loosely the DNA is wound. This, in turn, influences how accessible the genes are for expression.
Epigenetic Mechanisms: DNA Methylation, Histone Modification, and Non-coding RNAs
While DNA methylation and histone modification are the most well-studied mechanisms, they are not the only ones. Non-coding RNAs, particularly microRNAs and long non-coding RNAs, have recently emerged as significant players in the epigenetic orchestra. These molecules do not code for any proteins but can control gene expression by interacting with DNA, RNA, or protein molecules.
In essence, these epigenetic mechanisms allow for a dynamic response to the environment without changing the hard-coded genetic information. It is this flexibility that enables different cell types in our bodies to perform distinct functions despite having the exact same DNA sequence. It also allows cells to adapt and respond to various internal and external signals throughout life, playing crucial roles in development, learning, memory, and even in disease processes[4].
The Intersection of Neural Stem Cells and Epigenetics
Having established the foundational understanding of both neural stem cells and epigenetics individually, we are now poised to explore the intersection of these two intriguing fields. This crossroads presents a dynamic platform where the inherent plasticity of neural stem cells meets the modulating power of epigenetics. It is here that we find some of the most promising and exciting developments in neuroscience and regenerative medicine.
Epigenetic Control of Neural Stem Cell Fate
Epigenetic mechanisms play a central role in determining the fate of neural stem cells. DNA methylation, histone modification, and non-coding RNAs collectively orchestrate the gene expression patterns that guide these cells towards either self-renewal or differentiation.
For instance, specific DNA methylation patterns have been associated with maintaining stem cells in their undifferentiated state. Changes in these patterns can trigger differentiation, guiding neural stem cells to mature into neurons, astrocytes, or oligodendrocytes.
Similarly, histone modifications can change the structure of the chromatin, the material that makes up chromosomes, thereby influencing which genes are accessible and can be expressed. Finally, non-coding RNAs can fine-tune gene expression post-transcriptionally, offering yet another level of control over neural stem cell fate[5].
Epigenetic Modifications: Influencing Neural Stem Cell Differentiation
Epigenetic modifications not only influence whether neural stem cells self-renew or differentiate but can also guide the specific type of cell that they differentiate into. For example, particular histone modifications or DNA methylation patterns can push neural stem cells towards a neuronal or a glial fate.
The precise combination of epigenetic changes essentially provides a roadmap guiding the cell towards its ultimate identity. By studying these changes, researchers can gain insights into the molecular cues that drive neural development and regeneration.
Epigenetics and Neural Stem Cell Replication and Renewal
Epigenetic regulation is also critical for the replication and renewal of neural stem cells. As these cells divide, they must duplicate not just their DNA, but also their epigenetic information. Errors in replicating this epigenetic information can lead to changes in gene expression that may disrupt normal cell functioning and potentially lead to disease states. Therefore, understanding the mechanisms of epigenetic regulation during neural stem cell replication is crucial for harnessing the therapeutic potential of these cells[6].
Epigenetic Tools in Neural Stem Cell Research
The fusion of epigenetics with neural stem cell research has not only broadened our understanding of brain development and function, but also paved the way for the creation of innovative research tools. These tools allow scientists to manipulate epigenetic markers, offering unprecedented control over neural stem cell fate and function.
Unveiling Epigenetic Landscapes: Next-Generation Sequencing and Epigenomics
Unraveling the epigenetic control mechanisms governing neural stem cells begins with mapping their epigenetic landscapes. This involves identifying where on the DNA modifications like methylation occur, or understanding the specific patterns of histone modifications. Such mapping has been made possible through advancements in next-generation sequencing technologies and the development of the field of epigenomics, which is the study of the complete set of epigenetic modifications on the genetic material of a cell. By providing a comprehensive view of the epigenetic changes occurring in neural stem cells, these tools are helping us uncover the complex layers of regulation that dictate neural stem cell behavior.
Manipulating Epigenetic Markers: DNA Methyltransferases and Histone Deacetylases
Another exciting development in the field of epigenetic research is the ability to manipulate epigenetic markers. Enzymes like DNA methyltransferases, which add methyl groups to DNA, and histone deacetylases, which modify histone proteins, can be targeted to either add or remove epigenetic marks. These manipulations can influence the expression of specific genes, thereby controlling the fate and function of neural stem cells. By selectively turning genes ‘on’ or ‘off’, researchers can guide neural stem cells to renew themselves or differentiate into specific neural cell types[7].
Harnessing Non-Coding RNAs for Neural Stem Cell Research
The recognition of non-coding RNAs as key players in epigenetic regulation has added another tool in the neural stem cell research toolkit. MicroRNAs and long non-coding RNAs can be engineered and introduced into cells to influence gene expression patterns. This not only allows researchers to investigate the role of these molecules in neural stem cell biology but also offers a potential route for therapeutic intervention.
References
[1] Epigenetic Modulation of Stem Cells in Neurodevelopment
[2] Roles of epigenetics in the neural stem cell and neuron
[3] Epigenetic Regulations in Neural Stem Cells
[4] Selective translation of epigenetic modifiers affects the temporal pattern and differentiation of neural stem cells
[5] Epigenetic regulation of adult neural stem cells
[6] Neural stem/precursor cells dynamically change their epigenetic landscape
[7] Epigenetic Modifications of Stem Cells


