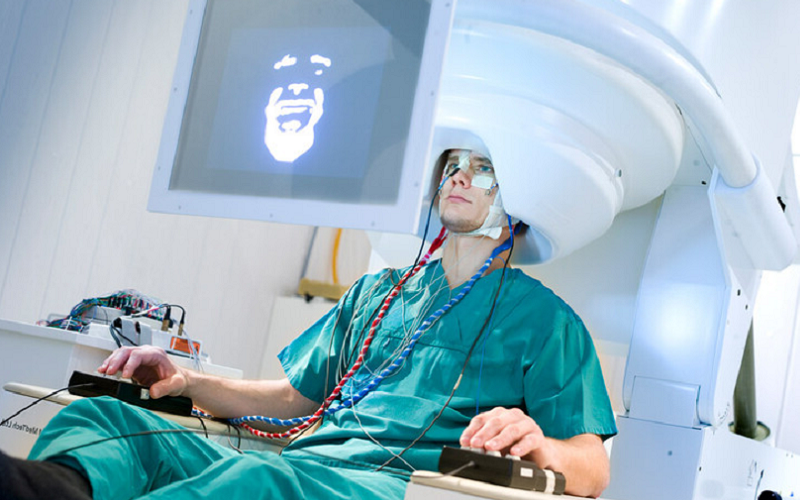
In the fascinating world of neuroscience, scientists constantly strive to decode the intricate web of neuronal activity that shapes our every thought, memory, and action. Among the various tools in our arsenal, one stands out for its extraordinary capacity to capture the brain’s dynamic dance in high resolution: Magnetoencephalography (MEG). This cutting-edge technology, with its roots in quantum mechanics, provides a non-invasive window into the brain, revealing invaluable insights about its functional organization in both health and disease.
Contents
Introduction to Magnetoencephalography (MEG)
Peering into the human mind has long been a quest for scientists, philosophers, and curious minds alike. Over the centuries, this quest has moved from mere philosophical musings to precise scientific endeavors. In this scientific journey, numerous tools and techniques have emerged, enabling us to delve into the depths of the human brain. Among these, one of the most innovative and exciting is Magnetoencephalography, commonly referred to as MEG.
Brief Overview of MEG
MEG is a non-invasive neuroimaging technique that measures the magnetic fields produced by neuronal activity in the brain. It allows scientists to capture brain signals with millisecond precision, providing an unparalleled window into the human brain’s workings. This remarkable capability has made MEG a fundamental tool in neuroscience, particularly in areas that require precise measurements of the brain’s activity in time and space.
The Importance of Studying Brain Dynamics
Human brain dynamics, or how different areas of the brain communicate and work together over time, play a crucial role in our cognitive functions. They underlie everything we do – from thinking and learning to sensing and moving. Studying these dynamics is essential not just for understanding the brain’s normal functioning but also for diagnosing and treating various neurological and psychiatric disorders. MEG, with its high temporal and spatial resolution, plays a vital role in this endeavour.
Historical Background of MEG
MEG’s roots trace back to the early 1960s, when the first evidence of magnetic fields associated with human brain activity was reported. The pioneering work of David Cohen in 1968, using a superconducting magnetometer, paved the way for modern MEG. Over the years, technological advancements, particularly the invention of the Superconducting Quantum Interference Device (SQUID), have allowed MEG to evolve into the sophisticated tool we know today. Despite being around for over half a century, MEG continues to push the boundaries of our understanding of the brain, leading us into an era of unprecedented insight into the mind’s inner workings [1].
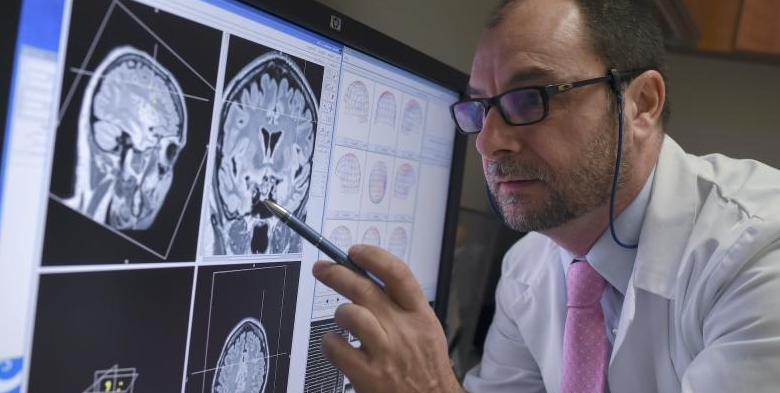
Understanding the Science Behind MEG
Before diving deeper into the world of MEG, it’s essential to understand the scientific principles that underpin this extraordinary technology. This will include exploring the basic principles of MEG, the crucial role played by Superconducting Quantum Interference Devices (SQUIDs), and how MEG compares with other neuroimaging techniques.
Basic Principles of MEG: From Magnetic Fields to Neural Activity
The science behind MEG is rooted in the fact that electrical currents produce magnetic fields – a principle known as Ampère’s circuital law. In the brain, when neurons fire, they generate small electrical currents. These currents, in turn, create tiny magnetic fields, which can be measured outside the head. By measuring these magnetic fields, MEG can indirectly detect the electrical activity of the brain.
The magnetic fields generated by neuronal activity are incredibly small, about a billion times weaker than the Earth’s magnetic field. This is where the remarkable sensitivity of MEG comes into play, allowing it to detect these minute fields and convert them into a comprehensible image of brain activity [2].
The Role of SQUIDs (Superconducting Quantum Interference Devices)
The secret to MEG’s sensitivity lies in a device called a Superconducting Quantum Interference Device, or SQUID. SQUIDs are the most sensitive magnetometers we have, capable of detecting even the tiniest changes in magnetic fields. When cooled to incredibly low temperatures, SQUIDs can pick up the minute magnetic fields generated by the brain’s electrical activity, making the invisible world of neuronal communication visible.
Comparison with Other Neuroimaging Techniques: EEG, fMRI, and PET
It’s worth noting that MEG is not the only tool at our disposal to explore the brain. Other prominent neuroimaging techniques include electroencephalography (EEG), functional magnetic resonance imaging (fMRI), and positron emission tomography (PET). While each of these has its strengths, MEG stands out for its exceptional temporal and spatial resolution.
Like MEG, EEG measures the electrical activity of the brain. However, unlike MEG, which measures the magnetic fields generated by this activity, EEG directly measures the electrical potentials. EEG is sensitive to the radial components of the electrical currents, while MEG captures their tangential components, offering complementary views of brain activity.
On the other hand, fMRI and PET measure changes in blood flow or glucose metabolism, which are indirect markers of neural activity. These techniques offer good spatial resolution but lack the temporal precision of MEG and EEG [3].
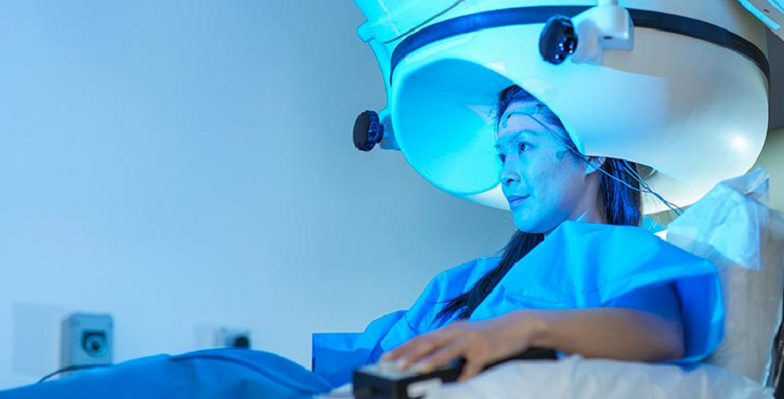
The MEG Procedure: What to Expect
Now that we have a grasp of the principles behind MEG, let’s delve into what to expect during an MEG scan. Like most medical procedures, an MEG scan involves three stages: preparation before the scan, the scan itself, and the interpretation of results after the scan.
Before the Scan: Preparation and Patient Safety
Preparation for an MEG scan is relatively straightforward. It’s crucial for patients to remove any metallic objects, such as jewelry, glasses, or hairpins, as these can interfere with the magnetic measurements. Patients with certain metallic implants or devices, such as pacemakers, may not be suitable candidates for MEG.
Before the scan, the patient’s head shape is digitized using a 3D digitizer. Small coils or markers may be placed on the scalp to serve as reference points during data analysis. In addition, the patient’s head position within the MEG helmet is tracked continuously during the scan to correct for any head movements [4].
During the Scan: The MEG Procedure Detailed
During the MEG scan, the patient sits or lies down in a comfortable position under the MEG device, which resembles a large helmet. It’s essential to stay as still as possible during the scan to ensure accurate measurements.
The MEG device does not produce any noise or cause any sensation, making the procedure comfortable for most people. The scan typically lasts for about an hour but can vary depending on the nature of the study. During the scan, the patient may be asked to perform certain tasks, such as viewing images or responding to stimuli, to elicit brain activity in specific regions.
After the Scan: Understanding the Results
Once the MEG scan is complete, the data undergoes complex processing and analysis. Sophisticated algorithms are used to convert the magnetic field measurements into a dynamic image of brain activity. This image can provide information about the timing and location of neural activity associated with specific tasks or stimuli.
The results of an MEG scan are typically reviewed by a team of experts, including neurologists, radiologists, and physicists, who interpret the complex data. The findings can be used for various purposes, from diagnosing neurological conditions to planning neurosurgery or informing research studies.
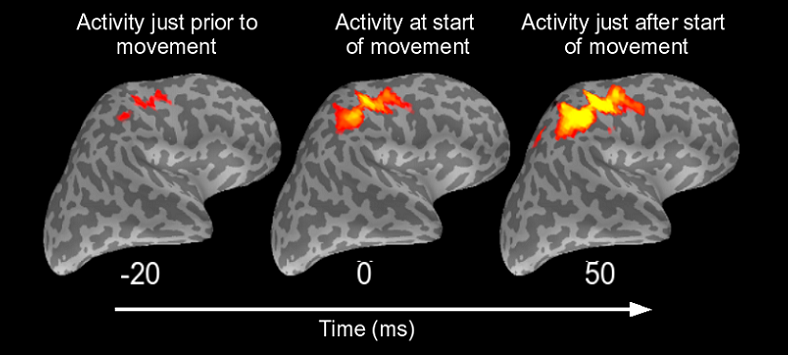
Applications of MEG in Neuroscience and Medicine
With its unique ability to provide a real-time, high-resolution view of brain activity, MEG has found wide-ranging applications in both neuroscience and clinical medicine. Whether it’s deciphering the brain’s response to a specific stimulus or aiding in the diagnosis of neurological disorders, MEG serves as a versatile tool in the hands of researchers and clinicians.
Cognitive Neuroscience: Studying Mental Processes
One of the key applications of MEG is in the field of cognitive neuroscience, where researchers are interested in understanding the brain processes that underlie our thoughts, emotions, and behavior. MEG provides a powerful way to study these processes in real time, shedding light on the dynamic interplay between different brain regions involved in various cognitive functions.
For example, MEG can be used to study how the brain processes sensory information, such as visual or auditory stimuli, how it orchestrates complex cognitive tasks like memory or attention, and how these processes may change in response to learning or aging. This wealth of information can help researchers paint a more complete picture of the human mind and its vast capabilities [5].
Clinical Diagnostics: Detecting Abnormal Brain Activity
MEG is not just a research tool; it also has important clinical applications. In medical settings, MEG is used to detect abnormal brain activity associated with various neurological disorders. For example, MEG can help localize the source of epileptic seizures in patients with epilepsy, providing valuable information that can guide treatment decisions.
Similarly, MEG can be used in the diagnosis and management of other neurological conditions like Alzheimer’s disease, Parkinson’s disease, and various psychiatric disorders. By revealing patterns of abnormal brain activity, MEG can help clinicians identify the presence and severity of these conditions, track their progression over time, and assess the efficacy of different treatments.
Advancements in Neurosurgery: Precise Mapping of Brain Functions
In the realm of neurosurgery, MEG serves a crucial role in pre-operative planning. It’s often vital for surgeons to identify and map out critical regions of the brain – such as those involved in language, sensory, or motor functions – before performing surgery. This process, known as functional mapping, helps surgeons avoid damaging these crucial areas during surgery, thereby minimizing post-operative complications.
MEG’s ability to provide precise spatial and temporal information about brain activity makes it an excellent tool for this purpose. By non-invasively mapping the brain’s functional regions, MEG can help neurosurgeons perform safer and more effective operations, improving patient outcomes [6].
Advantages of Using MEG
Given its diverse applications in neuroscience and medicine, it’s evident that MEG offers several advantages as a neuroimaging tool. From its superior temporal and spatial resolution to its non-invasive nature, MEG’s strengths enable it to provide unique insights into brain dynamics.
High Temporal and Spatial Resolution
One of the major advantages of MEG is its exceptional temporal resolution. With the ability to track changes in brain activity on the order of milliseconds, MEG can capture the brain’s fast-paced dynamics with remarkable precision. This temporal resolution is far superior to that of other imaging techniques like fMRI and PET, which measure slower metabolic processes rather than direct neural activity.
Alongside its temporal resolution, MEG also boasts excellent spatial resolution. It can localize the sources of neural activity within a few millimeters, allowing researchers and clinicians to pinpoint the brain regions involved in specific cognitive processes or exhibiting abnormal activity.
Non-Invasive and Safe Procedure
Another key strength of MEG is its non-invasive nature. Unlike procedures that require injections, implants, or exposure to radiation, MEG measures the brain’s natural magnetic fields without any physical intrusion or exposure to potentially harmful substances. This makes MEG a safe tool for studying brain activity, even in sensitive populations such as children or pregnant women.
Ability to Study Real-Time Brain Dynamics
Lastly, MEG’s ability to measure brain activity in real time offers a unique window into the brain’s dynamics. It can capture the sequence of neural events as they unfold, providing a dynamic view of the brain’s intricate dance. This capability is crucial for understanding the temporal coordination between different brain regions, which is fundamental to many cognitive processes [7].
Limitations and Challenges of MEG
While MEG is a powerful tool with many advantages, it’s also important to acknowledge its limitations and the challenges it presents. These range from practical considerations like cost and accessibility to more technical challenges related to data interpretation and analysis.
Cost and Accessibility
One of the significant limitations of MEG is its cost. MEG systems are expensive to purchase, install, and maintain, with costs often running into millions of dollars. This high cost is largely due to the need for sophisticated technology like SQUIDs and the shielding required to block out external magnetic fields.
In addition, MEG systems require special facilities and trained personnel to operate, further contributing to their expense. As a result, MEG is less widely available than other imaging techniques like EEG or fMRI, limiting its use primarily to research institutions and major medical centers.
Signal Interpretation and Source Localization
Another challenge with MEG lies in the interpretation of its signals. While MEG can measure the magnetic fields generated by neural activity with high precision, determining the exact sources of these signals within the brain – a process known as source localization – is a complex problem.
Multiple combinations of neural sources can produce the same magnetic field, making it difficult to definitively pinpoint the sources. While various computational methods have been developed to tackle this problem, it remains a significant challenge in MEG research.
Sensitivity to External Magnetic Fields and Patient Movements
MEG is also sensitive to external magnetic fields and patient movements. Since the magnetic fields generated by the brain are so tiny, they can easily be obscured by magnetic noise from the environment, such as electrical equipment or even the Earth’s magnetic field. Although MEG systems are typically housed in magnetically shielded rooms to minimize this issue, it remains a concern.
Similarly, movements by the patient during the scan, such as head movements, can distort the magnetic signals and complicate data analysis. Therefore, patients need to remain as still as possible during the scan, which can be challenging, particularly for certain populations like children or individuals with movement disorders.
References
[1] Magnetoencephalography (MEG): What It Is, Purpose & Uses
[2] What is Magnetoencephalography (MEG)?
[3] Magnetoencephalography
[4] Magnetoencephalography: Basic principles
[5] Magnetoencephalography (MEG)
[6] Basic Principles of Magnetoencephalography
[7] Magnetoencephalography: physics, techniques, and applications in the basic and clinical neurosciences




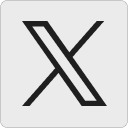




