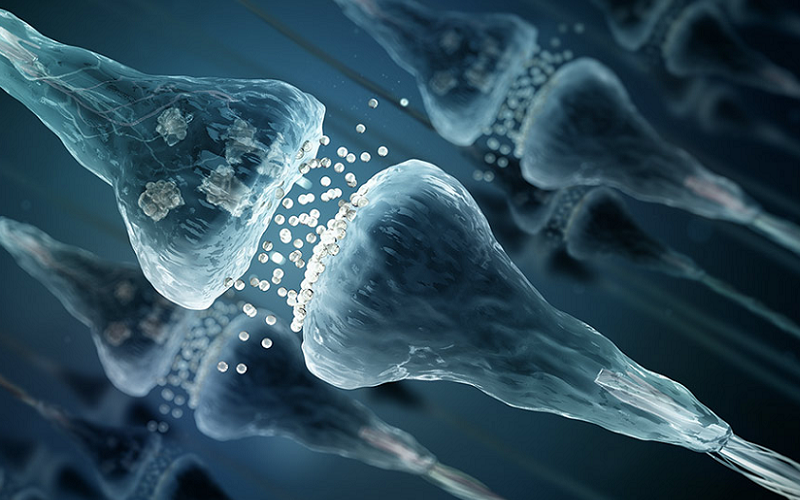
When it comes to the fascinating world of brain science, few concepts are as captivating — and crucial — as neuronal plasticity. Often dubbed the “cellular basis of learning and memory,” plasticity allows our brain to adapt and rewire itself in response to new experiences, from learning to play a musical instrument to mastering a new language. For years, much of the scientific discourse and research have focused on postsynaptic plasticity, mechanisms like Long-Term Potentiation (LTP) and Long-Term Depression (LTD) that alter the strength of connections after signals are sent across the synapse.
But what about the other side of the synapse? Enter presynaptic plasticity — the lesser-known counterpart that plays an equally significant role in how our neurons communicate and adapt. Just as pivotal but less publicized, presynaptic plasticity merits a spotlight for anyone interested in understanding the full scope of how our brain evolves and learns.
Contents
Introduction to Neuronal Plasticity
In neuroscience, one of the most enchanting subjects of study is neuronal plasticity — the brain’s remarkable ability to reorganize itself by forming new neural connections over time. This plasticity is the neural foundation behind our ability to learn, adapt, and even recover from brain injuries.
Importance of Plasticity in Learning and Memory
Imagine trying to navigate the world with a brain that’s hardwired and unchanging. It would be like using a map that never updates — pretty useless in a continually evolving landscape. That’s why neuronal plasticity is crucial. It’s what allows us to acquire new skills, remember faces, adapt to changes, and essentially, evolve. When you practice the piano every day or memorize mathematical formulas, it’s the plasticity of your brain that makes these accomplishments possible.
Brief Overview of Postsynaptic Plasticity
If you’ve ever dived into the topic of brain plasticity before, chances are you’ve come across terms like Long-Term Potentiation (LTP) and Long-Term Depression (LTD). These are forms of postsynaptic plasticity, changes that occur on the receiving end of a synaptic connection. They adjust the strength of neural pathways based on experience, thereby allowing the brain to store information. Postsynaptic plasticity has garnered much attention and is often what people think of when discussing the brain’s adaptability.
Introduction to Presynaptic Plasticity: The Lesser-Known Side
But there’s another side to this story that’s just as compelling and fundamental — presynaptic plasticity. This aspect of plasticity occurs at the transmitting, or presynaptic, side of the synaptic connection. In simpler terms, it’s all about how the sending neuron modifies its signaling. While postsynaptic plasticity focuses on the receiving end of the communication line, presynaptic plasticity is concerned with changes on the sending end. Yet, it hasn’t received as much spotlight as its postsynaptic counterpart.
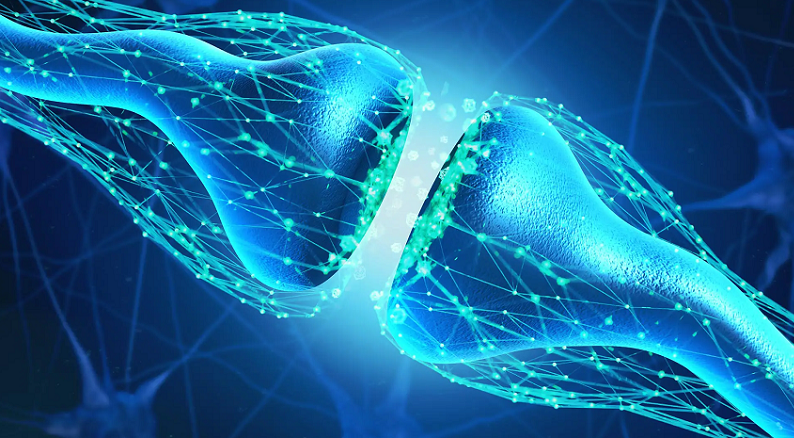
Understanding the Basics of a Neuron
Understanding the anatomy and physiology of a neuron will give us the foundation we need to comprehend more complex topics like presynaptic and postsynaptic plasticity. So let’s start by looking at the core components of a neuron.
The Anatomy of a Neuron
Cell Body
At the heart of every neuron is the cell body, also known as the soma. This is where the nucleus resides and where most of the neuron’s metabolic activities occur. Think of it as the ‘control center’ of the neuron. From the cell body, extensions called dendrites and axons emanate, which facilitate communication with other neurons.
Axon
The axon is the long, slender extension that carries electrical impulses away from the cell body toward other neurons or target cells. Encased in a fatty insulating layer known as the myelin sheath, axons facilitate the quick transmission of signals. At the end of the axon, you’ll find the axon terminals or presynaptic terminals, which are crucial for synaptic communication and the topic of this blog post.
Dendrites
In contrast to axons, dendrites are short, branched extensions that protrude from the cell body. Their primary function is to receive incoming signals from other neurons. These incoming signals often arrive in the form of neurotransmitters, which we will discuss later.
After exploring the building blocks of a neuron, let’s turn our attention to where neurons communicate with each other: the synapse.
The Synapse: Where the Action Happens
Presynaptic Terminal
The presynaptic terminal is the part of the neuron that releases neurotransmitters into the synapse. These neurotransmitters then travel across the synaptic cleft to bind to receptors on the receiving neuron. In essence, the presynaptic terminal is the ‘sending end’ of the synaptic dialogue and the main actor in presynaptic plasticity [1].
Synaptic Cleft
The synaptic cleft is the tiny gap that separates the presynaptic terminal from the postsynaptic membrane. It’s the ‘stage’ on which the exchange of neurotransmitters occurs. This gap might be minuscule—measuring just about 20 to 40 nanometers—but it’s a critically important space for neural communication.
Postsynaptic Membrane
The postsynaptic membrane is located on the ‘receiving’ neuron, typically on one of its dendrites. This membrane is studded with receptors that bind to neurotransmitters released by the presynaptic terminal. The postsynaptic membrane is central to postsynaptic plasticity, but it also plays a role in presynaptic plasticity through mechanisms like retrograde signaling.
Electrical and Chemical Signaling
Neurons communicate through a mix of electrical and chemical signals. Electrical impulses, called action potentials, travel down the axon to the presynaptic terminal. There, they trigger the release of neurotransmitters, the chemicals that carry the message across the synaptic cleft to the postsynaptic membrane.
These basic components and processes are the gears and pulleys behind the curtain of every thought, every movement, and every sensation you experience. Understanding these basics is crucial as we delve into more complex phenomena like presynaptic plasticity [2].
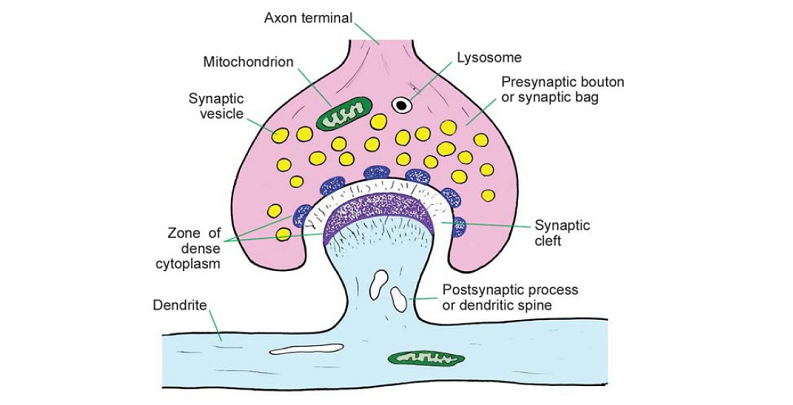
Postsynaptic Plasticity: The Well-Known Mechanism
Postsynaptic plasticity is the type of neural plasticity that has dominated scientific literature and popular understanding of how the brain adapts. You may already be somewhat familiar with this concept, but it’s worth revisiting. By comprehending postsynaptic plasticity, we can better appreciate the lesser-known but equally significant mechanisms of presynaptic plasticity.
Long-Term Potentiation (LTP)
What is LTP?
Long-Term Potentiation (LTP) is the persistent strengthening of synapses based on recent patterns of activity. It’s essentially the process that allows neurons to “remember” a particular stimulus or activity by enhancing their response to it. If you’ve heard the phrase “neurons that fire together, wire together,” that’s LTP in a nutshell.
How Does LTP Work?
LTP works through a variety of biochemical pathways, often involving an increase in the number or sensitivity of receptors on the postsynaptic membrane. This enhanced response enables a more efficient transfer of information across the synapse, making subsequent encounters with the same stimulus easier to process.
Long-Term Depression (LTD)
What is LTD?
Long-Term Depression (LTD) is the opposite of LTP; it’s a lasting decrease in synaptic strength between two neurons. This weakening is just as important as strengthening because it allows for the elimination of unnecessary or redundant connections. In essence, LTD is about neural “forgetting” and plays a crucial role in refining neural circuits.
How Does LTD Work?
LTD involves the removal or deactivation of postsynaptic receptors, making the synaptic connection less responsive to neurotransmitters. This process can help the nervous system remain flexible, allowing for the establishment of new and more relevant connections.
Role in Learning and Memory
Both LTP and LTD contribute significantly to the formation of memories and the acquisition of new skills. For instance, LTP might facilitate the retention of a new piece of information, such as a name or a date, by enhancing the neural pathway responsible for that memory. On the other hand, LTD could help you unlearn incorrect or outdated information by weakening the respective neural connections [3].
Limitations and Questions
Although postsynaptic plasticity has been widely studied, there remain gaps in our understanding. For instance, how specific are these processes? Do they target individual synapses or affect broader networks? Furthermore, there’s a growing realization that postsynaptic mechanisms alone can’t fully explain all forms of learning and memory, leading researchers to explore other avenues—like presynaptic plasticity.
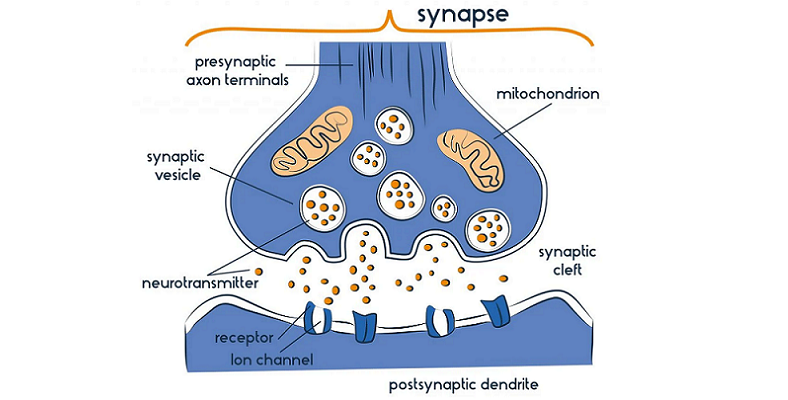
Introduction to Presynaptic Plasticity
Just as postsynaptic plasticity offers a framework for understanding how the brain changes on the receiving end of the synapse, presynaptic plasticity focuses on alterations that occur at the sending end. Despite its relative obscurity in mainstream discussions of neuroplasticity, presynaptic mechanisms are essential for a complete picture of how neurons adapt, learn, and communicate.
What Is Presynaptic Plasticity?
Definition and Importance
Presynaptic plasticity refers to the changes in the strength or efficiency of synaptic transmission that originate from the presynaptic neuron. It deals with alterations in how neurotransmitters are released into the synaptic cleft. Such changes can be both long-term and short-term and can lead to either an increase (potentiation) or a decrease (depression) in synaptic strength.
Differences from Postsynaptic Plasticity
While postsynaptic plasticity is often associated with modifications in receptor density or sensitivity on the receiving neuron, presynaptic plasticity involves changes in neurotransmitter release from the sending neuron. These might include alterations in the amount of neurotransmitter released, the probability of release, or the reuptake of neurotransmitters.
Forms of Presynaptic Plasticity
Short-Term Plasticity
Short-term presynaptic plasticity refers to transient changes in synaptic strength that can last from milliseconds to seconds. These rapid alterations can be either facilitatory, increasing the likelihood of neurotransmitter release, or inhibitory, decreasing it. These short-term changes are essential for processes such as sensory adaptation and temporal filtering of information.
Long-Term Presynaptic Plasticity
Long-term presynaptic plasticity involves more enduring changes in synaptic transmission. These can last from minutes to a lifetime and are crucial for long-term memory formation and the development of neural circuits. Like its postsynaptic counterpart, long-term presynaptic plasticity can also manifest as either potentiation or depression, enhancing or weakening synaptic connections over time.
Mechanisms of Presynaptic Plasticity
Presynaptic plasticity is governed by a plethora of cellular and molecular mechanisms. These might include alterations in calcium channel function, modifications in the availability of synaptic vesicles, or changes in the expression of specific proteins that regulate neurotransmitter release. Understanding these mechanisms can offer profound insights into not just how neurons communicate, but also how they adapt to changes in their environment or activity patterns [4].
Molecular Mechanisms Behind Presynaptic Plasticity
Having established what presynaptic plasticity is and its various forms, it’s time to examine the intricate molecular mechanisms that facilitate these changes. Understanding these processes is not just academic; it has significant implications for fields ranging from neuropharmacology to the study of neurodegenerative diseases.
Calcium Channels and Neurotransmitter Release
Role of Calcium Channels
One of the most critical components in presynaptic plasticity is the calcium ion (Ca2+) channel. When an action potential reaches the presynaptic terminal, voltage-gated calcium channels open, allowing an influx of Ca2+ ions. These ions act as a trigger for the release of neurotransmitters stored in synaptic vesicles.
Regulation of Calcium Influx
Regulation of these calcium channels is crucial for modulating neurotransmitter release. Changes in the number of active channels or their sensitivity can lead to variations in the amount of Ca2+ entering the terminal, thereby influencing neurotransmitter release. This regulation is a central mechanism for both short-term and long-term forms of presynaptic plasticity.
Synaptic Vesicles and Release Probability
Vesicle Pool Dynamics
Within the presynaptic terminal, neurotransmitters are stored in synaptic vesicles, which are tiny, membrane-bound compartments. These vesicles are divided into different ‘pools,’ based on their readiness to release neurotransmitters. Presynaptic plasticity often involves shifting vesicles between these pools, thereby affecting the probability of neurotransmitter release.
Regulation of Release Probability
The ‘readiness’ of a vesicle to release its contents upon receiving a signal is known as its ‘release probability.’ Factors like the proximity of vesicles to calcium channels and the availability of proteins that facilitate vesicle fusion can influence this probability. Through a variety of molecular pathways, the release probability can be increased or decreased, contributing to presynaptic plasticity.
Retrograde Signaling and Modulatory Factors
Retrograde Messengers
In some cases, the postsynaptic neuron can send signals back to the presynaptic neuron to modulate neurotransmitter release. These ‘retrograde messengers,’ which often include molecules like endocannabinoids or nitric oxide, play a pivotal role in regulating presynaptic function.
Modulatory Neurotransmitters and Receptors
Besides retrograde messengers, other modulatory neurotransmitters like dopamine and serotonin can also influence presynaptic plasticity. These neurotransmitters often bind to specific receptors on the presynaptic membrane, triggering intracellular pathways that ultimately affect neurotransmitter release.
As we can see, the molecular underpinnings of presynaptic plasticity are as complex as they are fascinating. From calcium channels to synaptic vesicles, and from retrograde messengers to modulatory neurotransmitters, a multitude of elements come together to enable the remarkable adaptability of presynaptic terminals.
Functional Implications of Presynaptic Plasticity
We’ve journeyed through the molecular intricacies of presynaptic plasticity, but the importance of these mechanisms is not confined to the molecular level. Understanding presynaptic plasticity offers profound insights into various functional aspects of the nervous system, from basic signal transmission to complex processes like learning, memory, and even the onset of neurological disorders.
Role in Learning and Memory
Facilitating Learning
Just as postsynaptic plasticity plays a critical role in the learning process, presynaptic plasticity contributes significantly to the adaptation and optimization of neural circuits during learning. Through modulation of neurotransmitter release, the presynaptic terminal can fine-tune the information flow, enabling more efficient learning processes [5].
Memory Storage and Retrieval
In addition to facilitating learning, presynaptic plasticity is crucial for the long-term storage and retrieval of memories. By selectively strengthening or weakening specific synapses, presynaptic mechanisms contribute to the stability and flexibility of memory networks.
Sensory Processing and Adaptation
Sensory Filtering
Presynaptic plasticity is integral to sensory adaptation and filtering. By modulating the release of neurotransmitters, the nervous system can adjust the sensitivity of sensory neurons to different stimuli. This allows the brain to focus on relevant information while filtering out the ‘noise.’
Neural Coding of Sensory Information
The presynaptic terminal plays a crucial role in the encoding of sensory information. By dynamically adjusting the release of neurotransmitters, presynaptic neurons can alter the pattern of postsynaptic firing, effectively ‘coding’ different types of sensory information in a more efficient manner.
Implications for Neurological Disorders
Neurodegenerative Diseases
Anomalies in presynaptic plasticity have been implicated in a variety of neurodegenerative diseases, such as Alzheimer’s and Parkinson’s. Understanding these presynaptic changes can provide insights into disease mechanisms and potential therapeutic targets.
Mental Health Conditions
Presynaptic mechanisms also have a role in mental health conditions like depression and anxiety. For instance, changes in the release or reuptake of neurotransmitters like serotonin and dopamine are critical aspects of these disorders, making presynaptic plasticity a potential focus for treatment strategies.
As we’ve seen, presynaptic plasticity has broad functional implications, affecting everything from our ability to learn and remember to our sensory perception and even our susceptibility to various neurological conditions. The next time you read about brain adaptability or neural learning mechanisms, remember that the story is not complete without considering the role of presynaptic plasticity.
References
[1] Presynaptic long-term plasticity
[2] Presynaptic long-term plasticity
[3] Synaptic Plasticity: Multiple Forms, Functions, and Mechanisms
[4] Presynaptic and Postsynaptic Mechanisms of Synaptic Plasticity
[5] Short-term forms of presynaptic plasticity

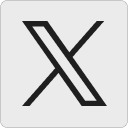





